Introduction: Launching Connectivity from the Tropics
In the world of space ventures, the smartest play isn't always the most obvious. While industry giants hurl billions at massive constellations aiming for instant global coverage, a different approach beckons—one that starts not with the poles, but with the waistband of our planet. The equatorial band, spanning roughly ±15° latitude, represents both an underserved market and a strategic launching point for a new generation of satellite networks.
This isn't just theoretical musing. Roughly two billion people live within this tropical belt, many in regions where terrestrial infrastructure struggles to keep pace with digital demands. Here, amid developing nations stretching from Ecuador to Indonesia, lies an opportunity to deploy a targeted constellation that delivers 5G Non-Terrestrial Network (NTN) connectivity where it's needed most—without the crushing capital requirements of a Starlink-scale operation.
The approach is elegantly pragmatic: start with a focused constellation serving the equatorial regions, generate revenue and operational experience, then expand outward in methodical phases until achieving global coverage. It's the difference between building a highway system one strategic corridor at a time versus attempting to pave every road simultaneously.
This isn't merely conservative—it's shrewd. By concentrating initial deployments where the Earth rotates fastest, satellites can leverage the planet's natural dynamics to maintain coverage with fewer units. The physics and economics align beautifully here, allowing a nimble operator to establish presence in markets typically overlooked by larger players fixated on North America and Europe.
What follows is a blueprint for this equatorial strategy—examining why conventional launch approaches fall short, why dedicated small launchers are transformative, how to calculate an effective constellation size, and the phased expansion path from tropical belt to global service. For investors seeking asymmetric opportunities in NewSpace that don't require ten-figure commitments, this represents a flight plan worth considering.
The Rideshare Launch Illusion (and Why It Falls Short)
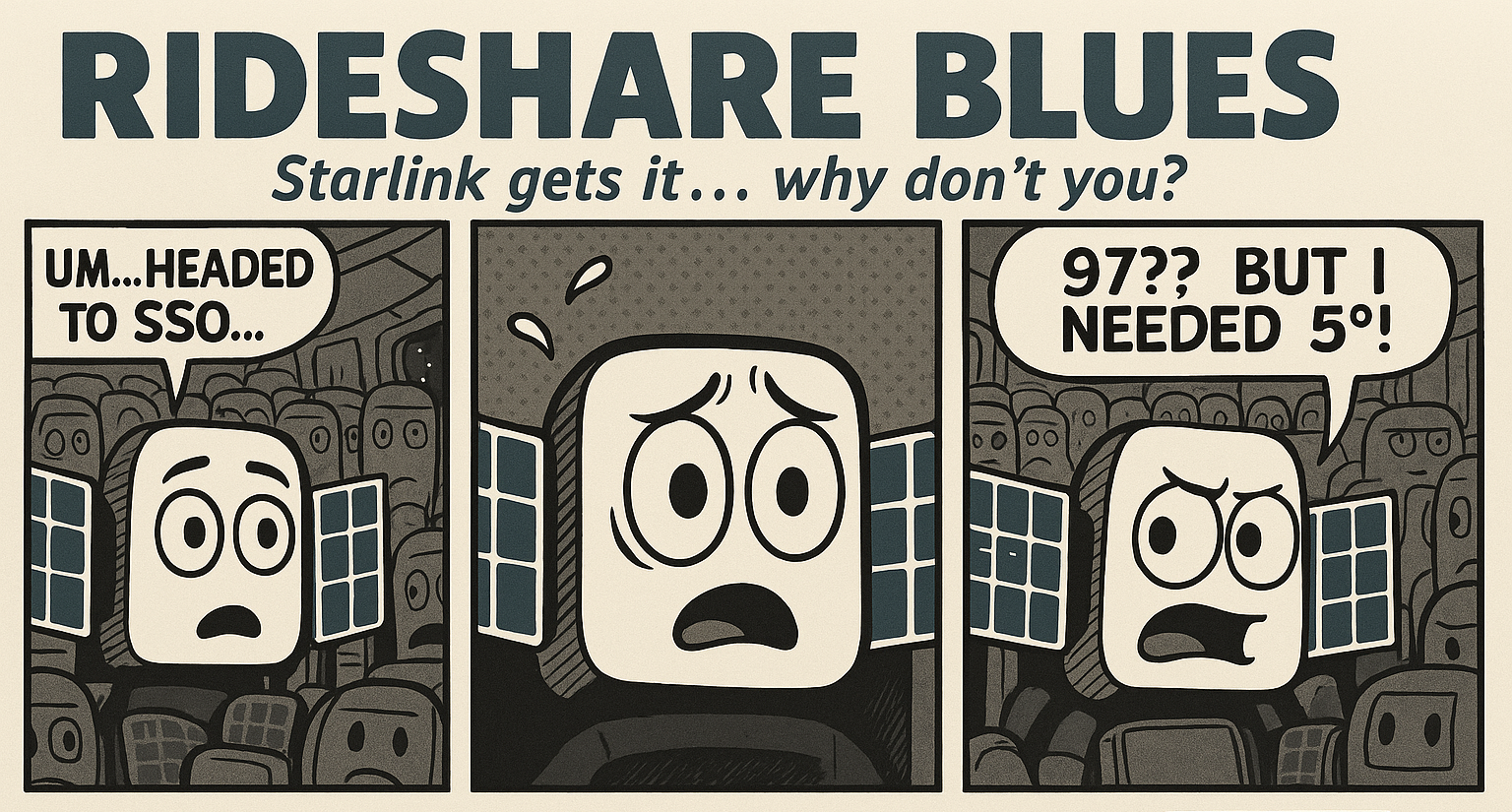
The appeal is undeniable. Book a slot on someone else's rocket, pay a fraction of the full launch cost, and your satellite reaches orbit without the hassle of procuring a dedicated vehicle. For university cubesats and technology demonstrators, this carpooling approach to space has democratized access to orbit in revolutionary ways. The numbers tell the story: roughly $30,000 per kilogram fully integrated versus $50,000+ for dedicated launches.
But constellations are a different animal entirely. They don't just need to reach space—they need to reach specific orbits at specific times in a carefully orchestrated sequence. This is where the rideshare model breaks down with the predictability of orbital mechanics itself.
Consider the constraints:
Orbital Inflexibility: Rideshare passengers don't choose their destination—they accept whatever orbit the primary customer requires. Need an equatorial inclination of 5° to optimize coverage over Indonesia? That's lovely, but the rideshare is headed to a 97° sun-synchronous orbit because the primary Earth-observation payload requires it. The mismatch is fundamental and unresolvable.
Schedule Dependency: Constellation deployment requires cadence and certainty. With rideshares, you launch not when your network planning dictates, but when a seat becomes available on someone else's flight. The resulting timeline uncertainty makes network planning nearly impossible and extends the capital-intensive period before service revenue can begin.
Deployment Clustering: Even after reaching orbit, rideshare-deployed satellites emerge as a tight cluster, requiring months of careful propulsive maneuvering to reach their designated positions around an orbital plane. For smallsats with limited propellant budgets, this positioning phase consumes precious resources that could otherwise extend operational lifetime.
Limited Destination Options: The vast majority of rideshare missions target either sun-synchronous orbits (97-98° inclination) or mid-inclination orbits around 45-55°. If your constellation architecture calls for equatorial positioning, you'll find these opportunities exceedingly rare. You'll either compromise your orbital design or carry substantial extra propulsion, negating the cost advantage that made rideshare attractive initially.
Constellation Coordination Challenges: A functional constellation isn't simply a collection of satellites—it's a carefully arranged system where spacing, phasing, and orbital parameters are precisely calculated to ensure consistent coverage. Rideshare deployments make this precision nearly impossible, creating a system with inherent coverage gaps and service inconsistencies.
The hard truth is that constellation architecture and rideshare economics exist in fundamental tension. Planet Labs' approach worked precisely because their "always-on" imaging model could tolerate gaps and imperfect spacing. Communication constellations, where continuous coverage is the core value proposition, simply cannot afford this compromise.
SpaceX grasped this early. Despite owning rockets and theoretically having the option to deploy Starlink satellites as secondary payloads, they chose dedicated launches because constellation integrity demanded it. The lesson is clear: if the company whose founder revolutionized reusable rocketry still opts for dedicated launches for their constellation, smaller players should take note.
Dedicated Micro-Launchers: Launch on Your Own Terms
While SpaceX solved their constellation deployment needs with their own vehicles, smaller operators face a different calculus. Traditional medium-lift launchers like Falcon 9 or Soyuz can carry dozens of satellites but come with price tags approaching $70 million—far beyond the reach of most startups. This gap between rideshare limitations and large rocket costs has created the perfect niche for a new class of vehicles: dedicated small launchers.
These purpose-built rockets deliver precisely what constellation operators need: orbital specificity, scheduling control, and right-sized capacity—all at costs that, while higher per kilogram than rideshare, enable the strategic deployment patterns essential for network integrity. Several players are now emerging in this critical market segment:
Isar Aerospace: Germany's leading private launch company is developing their Spectrum rocket, capable of lifting up to 1,000 kg to low Earth orbit. With backing exceeding €150 million from investors including Porsche SE and Airbus Ventures, Isar represents Europe's most advanced small launcher effort. Their first demonstration flight is scheduled for late 2025, with commercial services beginning in 2026.
Vaya Space: Taking an innovative approach to propulsion, this Florida-based company utilizes a hybrid rocket system that employs recycled thermoplastics as fuel. Their Symphony vehicle aims to carry up to 600 kg to LEO with first commercial flights targeted for mid-2026. Their proprietary STAR-3D™ fuel technology promises both cost advantages and environmental benefits compared to traditional solid or liquid systems.
The economics reveal why these vehicles matter. A dedicated small launcher might cost $15-20 million for a mission carrying 600-1,000 kg to orbit. While this translates to a higher per-kilogram cost than rideshare ($20,000-30,000/kg versus $10,000-15,000/kg), it delivers what constellation operators truly need: precision orbital insertion, launch timing control, and the ability to deploy satellites directly into their operational positions.
For our equatorial constellation scenario, these capabilities translate to tangible advantages:
- Direct deployment into low-inclination orbits that are rarely served by rideshare missions
- Ability to schedule launches to coincide with constellation expansion needs
- Precision insertion that minimizes the propellant satellites must use for positioning
- Flexibility to replace failed units or augment coverage in high-demand regions
This shift in launch paradigm—from opportunistic hitchhiking to strategic deployment—represents a crucial enabling factor for our equatorial-first network strategy. But the question remains: how many satellites do we actually need to cover the tropical band where potential users await service?
Sizing the Equatorial Mini-Constellation
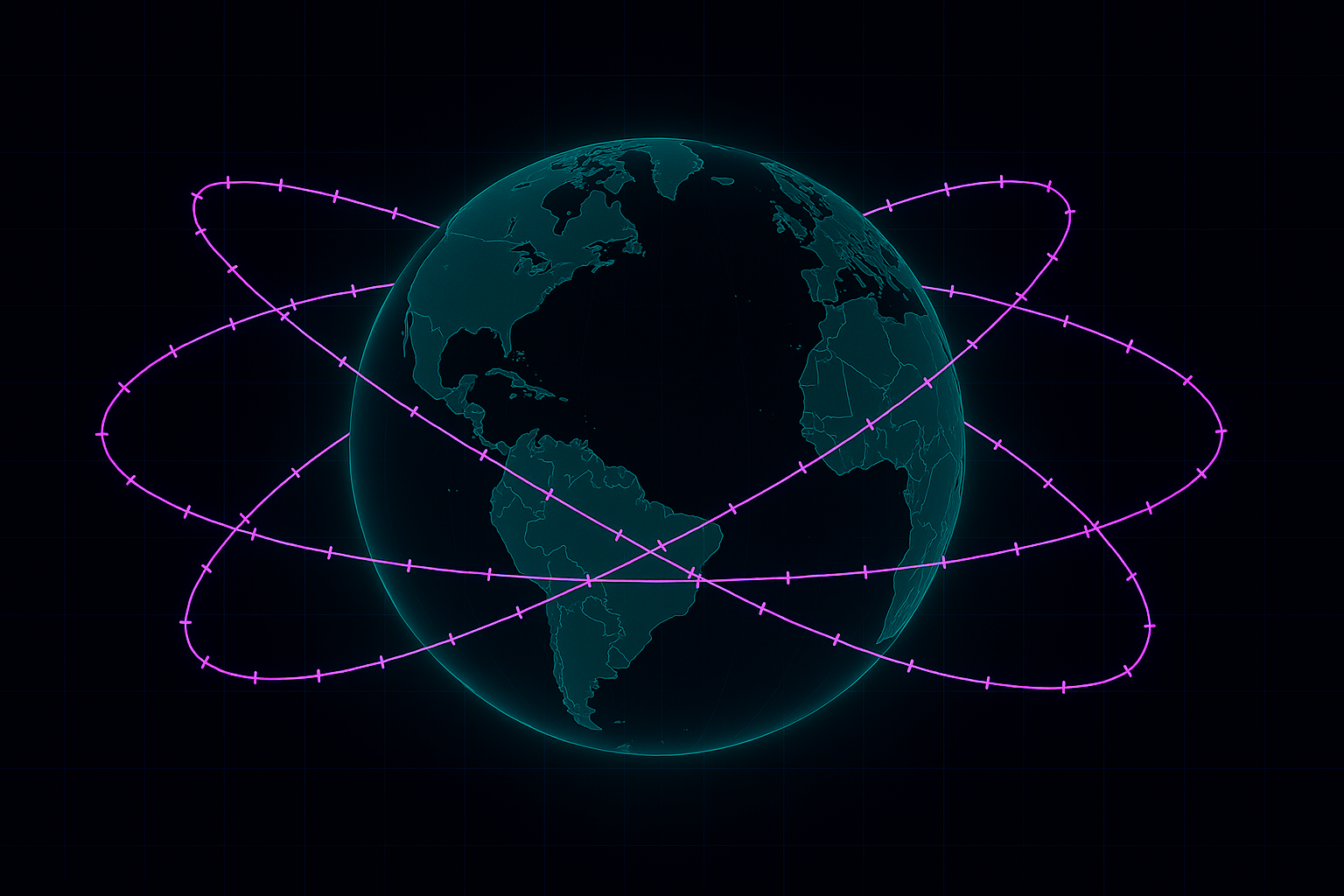
To determine the optimal constellation size for an equatorial 5G-NTN service, we must work backward from service requirements. Unlike global constellations that require hundreds or thousands of satellites, an equatorial focus allows us to achieve meaningful coverage with a surprisingly modest fleet.
The physics works in our favor here. Satellites orbiting along the equator benefit from the Earth's wider diameter at this latitude, covering more territory than their polar counterparts during each orbital pass. Additionally, service focused on the ±15° latitude band means we're only concerned with covering about 26% of Earth's surface—not the poles or high-latitude regions where other constellations concentrate their capacity.
Let's run the calculations
For 5G-NTN service with reasonable latency, we'll target a Low Earth Orbit altitude of 600 km. At this height, each satellite has a coverage footprint approximately 2,500 km in diameter when projecting to Earth's surface (assuming a minimum elevation angle of 30° for quality service).
At the equator, Earth's circumference is 40,075 km. Dividing this by our satellite footprint diameter gives us the minimum number of satellites needed in a single orbital plane to ensure continuous coverage: 40,075 ÷ 2,500 ≈ 16 satellites.
However, this assumes perfect spacing and doesn't account for handover requirements or the need for some overlap between coverage zones. Adding a 20% buffer brings our single-plane requirement to approximately 19-20 satellites.
To extend coverage to the full ±15° latitude band, we need additional orbital planes. Given the width of this band (approximately 3,300 km north-to-south), and our satellite footprint diameter of 2,500 km, a minimum of two additional orbital planes would provide coverage across the entire target region.
Thus, our minimum viable constellation becomes:
3 orbital planes × 20 satellites per plane = 60 satellites
This represents the theoretical minimum for continuous coverage. In practice, a more robust initial deployment would likely include:
4 orbital planes × 20 satellites per plane = 80 satellites
This configuration provides several advantages:
- Redundancy against individual satellite failures
- Higher capacity in high-demand areas
- Improved elevation angles that enhance service quality
- Some coverage extending beyond the strict ±15° band into adjacent markets
For comparison, this is less than 5% of Starlink's current deployment of over 1,700 satellites, yet could deliver service to regions containing nearly two billion potential users—a focused approach that prioritizes capital efficiency.
Equatorial User Base: Not So Niche After All
The equatorial strategy isn't merely about easier constellation deployment—it targets a substantial and underserved market. When we overlay population density with the ±15° latitude band, the opportunity becomes clear.
This tropical belt encompasses:
- Portions or all of 72 countries across Africa, South/Southeast Asia, and Latin America
- Approximately 2 billion people, or roughly 25% of the global population
- Some of the world's fastest-growing economies and digital adoption rates
- Regions where terrestrial infrastructure deployment faces significant geographic challenges
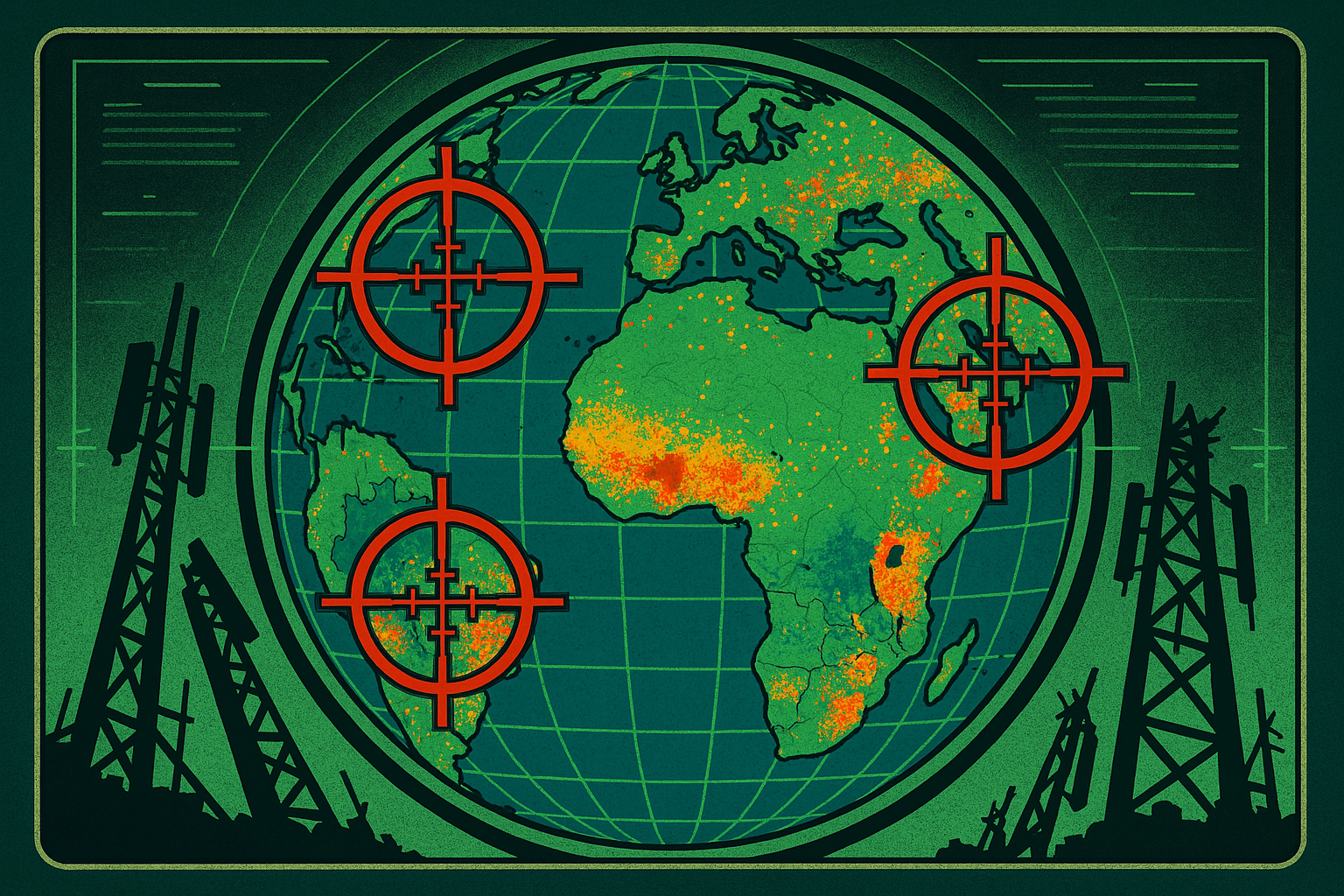
Key markets within this band include:
- Indonesia (273 million people)
- Brazil (212 million people)
- Nigeria (206 million people)
- Pakistan (partial coverage, 220 million people)
- Mexico (partial coverage, 126 million people)
- Philippines (109 million people)
- Vietnam (97 million people)
- Ethiopia (115 million people)
- DR Congo (90 million people)
- Thailand (70 million people)
Critically, these regions also exhibit some of the largest gaps in broadband penetration. While North America and Europe enjoy broadband penetration rates exceeding 80%, many countries in the equatorial band have rates below 30%. The connectivity gap represents not just a market opportunity but a chance to drive meaningful economic development through improved digital access.
Within this population, our target market segments include:
- Rural communities beyond the reach of fiber or 4G/5G terrestrial networks
- Maritime users in the heavily trafficked shipping lanes crossing the equatorial Pacific, Indian Ocean, and Atlantic
- Resource extraction operations (mining, forestry, oil & gas) operating in remote tropical regions
- Disaster recovery in regions prone to earthquakes, typhoons, and flooding that disrupt terrestrial infrastructure
- Government and security services requiring assured communications
Even capturing just 1% of the addressable population would yield 20 million subscribers—a substantial user base capable of supporting a profitable operation without requiring the global scale of larger constellation efforts.
Phased Expansion: From Equator to Global
The equatorial-first approach doesn't preclude eventual global coverage—it simply reorders the deployment sequence to prioritize early revenue and operational maturity. Once the equatorial constellation demonstrates commercial viability, expansion can proceed methodically:
Phase 1: Equatorial Belt (±15°)
- 80 satellites in 4 planes
- Coverage for 2 billion potential users
- Operational milestone: Positive cash flow
Phase 2: Mid-Latitude Expansion (±30°)
- Additional 160 satellites in 8 planes
- Coverage extended to include major markets in Mexico, India, Egypt, Australia
- Operational milestone: Sustained profitability and proven service model
Phase 3: High-Latitude Coverage (±50°)
- Additional 240 satellites in 12 planes
- Coverage of most populated regions including Europe, China, and North America
- Operational milestone: Established competitive position in global market
Phase 4: Global Coverage (±75° and beyond)
- Final 120 satellites in 6 planes
- Complete global coverage including polar regions
- Operational milestone: Full-spectrum global service provider
This phased approach allows capital deployment to be matched with demonstrated market traction. Each expansion builds upon validated technology and operations rather than requiring the full global system to be financed upfront. The total system (600 satellites) remains substantially smaller than Starlink's planned 12,000+ constellation, yet provides comprehensive global coverage for 5G-NTN applications.
Technical Parameters: The Nuts and Bolts
While the business strategy of equatorial-first deployment offers capital efficiency advantages, the technical implementation must be sound to deliver reliable service. Several key technical considerations shape the system architecture:
Orbital Parameters:
- Altitude: 600 km circular orbits
- Inclination: 5° for equatorial planes
- Spacing: 18° separation between satellites in same plane
- Planes: 90° separation in Right Ascension of Ascending Node (RAAN)
Satellite Design:
- Mass: 150-200 kg
- Power: 1.2 kW solar array with 3.5 kWh battery capacity
- Dimensions: 1m × 1m × 0.5m (stowed)
- Propulsion: Electric propulsion system for orbit maintenance (150 m/s ∆v capacity)
- Design life: 5-7 years
Communications Payload:
- 5G NR technology compatible with 3GPP Release 17 NTN standards
- Frequency bands: n257 (28 GHz) and n258 (26 GHz) for downlink/uplink
- Multiple spot beams: 18-24 beams per satellite
- Capacity: 20 Gbps aggregate throughput per satellite
- Ground terminal compatibility: Modified 5G smartphones and dedicated CPE devices
Inter-Satellite Links:
- Optical crosslinks between adjacent satellites in same plane
- Ka-band RF links between planes
- Mesh architecture enabling traffic routing within constellation
- Latency: <50 ms for equatorial coverage, progressively higher for expanded phases
Ground Segment:
- Gateway locations: Ecuador, Kenya, Indonesia, Marshall Islands, Brazil, Ghana
- Redundant fiber connectivity to internet exchange points
- Distributed cloud architecture for core network functions
- Edge computing capability for latency-sensitive applications
These technical parameters enable a system that balances performance, cost, and deployment complexity. The architecture allows for incremental improvements in satellite capabilities across deployment phases, incorporating lessons learned and technology advancements.
Regulatory Landscape: Navigating the Spectrum Maze
Any satellite constellation must navigate complex regulatory environments, and our equatorial approach is no exception. However, focusing initially on equatorial nations offers certain regulatory advantages worth noting:
Spectrum Allocation:
- Many equatorial nations have less congested millimeter wave spectrum compared to North America and Europe
- Opportunity to secure early spectrum rights in growing markets
- Potential to partner with national telecom authorities as part of digital inclusion initiatives
Landing Rights:
- Equatorial nations often have streamlined processes for new telecommunications services
- Possibility to obtain market access through partnership with incumbent operators
- Digital development initiatives in many countries create policy alignment with satellite broadband goals
ITU Filings:
- Equatorial focus allows for more specific and limited initial filings
- Phased approach aligns with ITU's progressive implementation requirements
- Opportunity to coordinate with fewer existing networks compared to polar regions
National Security Considerations:
- Lower regulatory barriers regarding security reviews in many equatorial markets
- Opportunity to develop partnerships addressing digital sovereignty concerns
- Early mover advantage in establishing security standards and practices
The regulatory strategy would emphasize partnership with host nations rather than pursuing a unilateral deployment model. By positioning the constellation as critical infrastructure supporting national development goals, the project can align with governmental priorities and potentially access development financing mechanisms.
Competitive Differentiation: Finding the Blue Ocean
The satellite communications market is increasingly crowded, but the equatorial-first strategy carves out a distinctive position. Several factors create sustainable competitive advantages:
Geographic Focus:
- Concentrated coverage where other constellations have diluted capacity
- Optimization for equatorial needs versus systems designed primarily for northern markets
- Higher elevation angles in target regions, improving urban canyon and foliage penetration
5G Integration:
- Direct compatibility with terrestrial 5G networks
- Ability to function as supplementary coverage for existing mobile operators
- Seamless handover between satellite and terrestrial infrastructure
Market Approach:
- Focus on underserved high-growth markets
- Partnerships with local telecom providers rather than direct competition
- Integration with regional development initiatives and financing
Cost Structure:
- Lower capital intensity than global constellations
- Faster path to revenue generation
- Ability to scale in response to demonstrated demand
While Starlink, Kuiper, and OneWeb pursue global coverage with emphasis on developed markets, our approach targets the equatorial opportunity gap—regions where terrestrial infrastructure lags but digital adoption is accelerating rapidly. This creates the possibility of establishing strong market position in high-growth regions while larger players focus their resources elsewhere.
Conclusion: The Case for Equatorial First
The space industry has historically oscillated between grand visions requiring massive capital and more incremental approaches that often lack transformative potential. The equatorial-first constellation strategy represents a pragmatic middle path—substantial enough to deliver meaningful service improvement across regions containing billions of potential users, yet focused enough to achieve commercial viability without requiring ten-figure investments before seeing the first dollar of revenue.
For investors, this approach offers several compelling advantages:
- Capital Efficiency: Requiring approximately $520M for initial deployment versus multi-billion dollar commitments for global systems
- Earlier Revenue: Service beginning with just 40 satellites (half the full equatorial constellation)
- Focused Market: Addressing specific high-need regions rather than competing immediately in saturated markets
- Expansion Optionality: Ability to scale based on demonstrated success rather than speculative forecasts
- Exit Flexibility: Creates multiple potential exit paths through regional telecom partnerships, acquisition by global players seeking market entry, or continued independent growth
The equatorial strategy also aligns with broader trends in space investment, where targeted approaches addressing specific market needs are increasingly demonstrating superior returns compared to all-encompassing platforms requiring massive scale to achieve viability.
For those seeking asymmetric opportunities in NewSpace, the path might just run along the equator—where physics, market demographics, and capital efficiency converge to create a distinctive opportunity for the next generation of satellite communications.